Concrete-faced rockfill dams (CFRD) have been the preferred dam type for high embankment dams for almost fifty years. They are claimed to be the safest dam type by their proponents. Here, Martin Wieland discusses the vulnerabilities of CFRDs and provides a comparison with earth core rockfill dams (ECRD) – the dam type which was replaced by CFRDs, writes Martin WIELAND, Dr. sc. techn., Chairman of ICOLD Committee on Seismic Aspects of Dam Design, Dam Consultant, Dietikon, Switzerland and AFRY Switzerland, Zurich, Switzerland
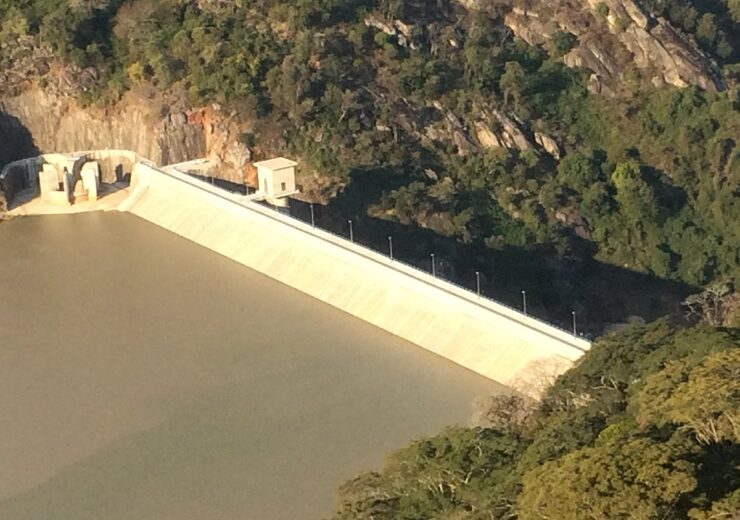
The Tokwe Mukosi Dam is a concrete-face rock-fill dam in Zimbabwe. (Credit: Tariro Mangena/Wikimedia Commons)
In recent years concrete-faced rockfill dams (CFRDs) have been built in increasing number as an economic alternative to conventional earth-core rockfill dams (ECRDs). Several of these dams already completed or under construction have heights exceeding 150m. CFRDs are also built in seismically active regions. They have been claimed to be the safest dam type by their developers. Today, such claims are also made for asphalt core rockfill dams. The two basic failure modes of embankment dams are progressive erosion due to overtopping and internal erosion.
Protection against overtopping can be provided by an adequate freeboard, by widening and strengthening the crest, by wave walls on the crest, etc. In composite dams consisting of a concrete section and embankment sections or dams with saddle dams, the overtopping risk of the embankment dam and the saddle dams, which are usually embankment dams themselves, can be reduced by increasing their height so that in the worst case the concrete section of a composite dam will be overtopped dam, but not the vulnerable embankment dams.
Maximum overtopping depths of up to about 2m may be accepted for concrete dams. However, the sliding stability of overtopped dams must be checked. This may be a safety problem for small gravity dams, but usually not for high concrete dams. In storage schemes comprising a main dam and saddle dams, one of the saddle dams could be used as a fuse plug or emergency spillway and the crest elevations of the other dams forming the reservoir should be higher than that of the fuse plug.
Using adequate filters embankment dams can be protected against internal erosion. Due to seismic deformations or slope failure the filter may be damaged. Dams with thin filters, whereby many of them are older dams, are vulnerable to such deformations.
As dams may be subjected to many different hazards from the natural and man-made environments as well as site-specific and project-specific hazards (Wieland, 2023), which may affect dams in different ways, it is too soon to maintain that certain dam types are safer than others. For example, a comparison of different dam types (CFRD, ECRD, gravity dam and arch dam) subjected to the multiple hazards associated with strong earthquakes, i.e. ground shaking, movements of faults or discontinuities in the footprint of dams and rockfalls at the dam site, was made by Wieland (2016). This qualitative comparison showed that for the abovementioned seismic hazards the best solution would be a conventional ECRD, as CFRDs would be vulnerable to fault movements, rockfalls on the face slab and ground shaking due to the different deformational and strength properties of the concrete face and the rockfill. However, the damage to the face slab due to rockfalls could be prevented by rockfall nets and other protective measures and the problems caused by the different deformational behaviour of the concrete face and the rockfill could be overcome by using rockfill with a high modulus of elasticity and by providing adequate vertical and horizontal joints in the face slab.
Because of the high permeability of rockfill, CFRDs should not fail if leakage through the face slab occurs, which also applies to asphalt core rockfill dams. However, in order to achieve a high stiffness of the rockfill, the grain distribution must include a fraction of relatively fine particles, which influences the permeability of the rockfill and a water table may develop near the base of the dam, depending on the amount of leakage through the concrete face, i.e. mainly through the joint sealing system.
The primary objective of this paper is to discuss the main factors, which could damage the concrete face, the joints between concrete slabs, waterstops and the plinth, and to provide recommendations on how these damages may be prevented or minimized.
One must also keep in mind that like other dams, CFRDs are prototypes, therefore generalizations are difficult and the damage that could occur in one dam may not occur in another one.
Failure or near-failure of CFRDs
The performance of CFRDs have been discussed by Marulando and Marulando (2008). They listed several high CFRDs, in which damage occurred in face slabs. Based on these observations, improvements were made in the design of face slabs and the water proofing system of the joints, which also account for larger joint movements as experienced, e.g. under seismic action.
Up to now the following CFRDs have failed: (i) Gouhou CFRD in China due to seepage and internal erosion (Zhang and Chen, 2006) and (ii) Taum Sauk CFRD in the US due to overtopping (ASDSO, 2005).
Gouhou Dam, a 71m high concrete-faced rockfill dam with a crest length of 265m, which was completed in 1990 in Qinghai Province, China, stored a reservoir with a volume of 3.1Mm3. Reservoir impoundment started during the construction of the dam and when it was completed the reservoir level was 3.9m below the normal water level and seepage flow was observed in the downstream slope. When the reservoir level reached a maximum on August 27, 1993, seepage increased and caused failure of the dam. About 320 people were killed due to the catastrophic release of the reservoir water. The main reasons for this incident were the leakage through the horizontal joint of the concrete face and the parapet wall structure on the dam crest, and rockfill materials that were susceptible to internal erosion.
The embankment dam forming the upper reservoir of the Taum Sauk Pump Storage Plant in Missouri, US, completed in 1962 and failed on December 14, 2005, due to pumping when the reservoir was full, causing overtopping of the dam and progressive erosion and dam failure. Fortunately, nobody was killed in the flood wave resulting from the dam`s failure, but the damage was estimated at US$1 billion.
The embankment dam of the Upper Reservoir, was made of dumped, uncompacted rockfill. The inside of the reservoir was lined with shotcrete. In 2004, a geomembrane liner was installed to reduce the leakage through cracks in the shotcrete caused by differential settlements (ASDSO, 2005). Because this shotcrete liner was the water proofing element of this dam it is considered to be a type of CFRD, although this face slab has little in common with that of the CFRDs built today.
The main reasons for this dam failure were deficient water gauges, so that the operator was not aware that the reservoir was full and with the absence of any spillway. Although there was no inflow into the upper reservoir, a spillway with a minimum discharge capacity equal to the maximum pump capacity would be needed.
The failure of the Gouhou CFRD may be considered as representative for the internal erosion failure mode and the failure of the Taum Sauk CFRD as representative for the overtopping failure mode.
In 2014 the 90m high Tokwe Mukosi CFRD in Zimbabwe, which stores a reservoir with 1.75km3, nearly failed during a severe flood in February 2014, when the dam was still under construction and the upstream concrete face was not yet built. Severe seepage occurred as no waterproofing membrane was in place.
With some delays the dam was completed and was commissioned in 2017. This important case study shows that excessive seepage through the rockfill can endanger the safety of CFRDs and that they may not be inherently safe as claimed by the designers of these dams. Of course, this also applies to rockfill dams with other types of upstream membranes, such as asphaltic concrete membranes or geomembranes or asphalt core rockfill dams.
Regarding the load bearing behaviour of CFRDs or other dams with an upstream waterproofing membrane, the transfer of the water load to the foundation rock is much more efficient than in the case of ECRDs, asphalt core rockfill dams or dams with a concrete core wall.
The main advantage of CFRDs is the significantly smaller dam volume than a comparable ECRD as the upstream and downstream dam slopes are generally steeper than those of ECRDs. ECRDs also require large quantities of core materials, which may not be readily available at some sites.
However, in this paper, the focus will be on safety aspects of CFRDs and the weakest element in CFRDs is the concrete face, which must be watertight. In this discussion, the benchmark will be conservatively designed CFRDs, which may be considered to be the most resilient embankment dam type. But we must keep in mind that all embankment dams are vulnerable to overtopping and, therefore, as a prerequisite these dams must have adequate flood discharge outlets. Today, the safety flood for embankment dams is the PMF (probable maximum flood), which must be released under the following assumptions:
- The power plant is out of operation, i.e. no discharge through power waterways.
- The outlet (spillway opening or low-level outlet) with the largest discharge capacity is closed. In dams with several spillway openings more than one opening has to be assumed to be closed.
- The maximum water level in the reservoir shall not exceed the top of the impervious core of ECRDs. For CFRDs the reservoir level may be up to the dam crest. Special criteria may apply to account for waves in the reservoir and storm surges in large reservoirs.
The present discussion also applies to saddle dams, whose failure has similar consequences to the failure of the main dam. If the same safety criteria have to be applied to the levees of run-of-river power plants as for saddle dams, this is not addressed in this paper because levees may be considered to be a kind of saddle dam.
We must also recognize that a dam with a long economic and safe life requires a good design, good quality of all construction works, regular maintenance and continuous safety monitoring.
Hazards affecting CFRDs
The hazards affecting the safety of all types of dam were discussed by Wieland (2023). The main hazards relevant for CFRDs can be classified as follows:
1. Natural hazards:
- Flood hazard (overtopping of dam, sedimentation of reservoir or blocking of spillway by floating debris).
- Earthquake hazard (inelastic deformations of dam body due to ground shaking or deformations in the dam footprint).
- Mass movement at dam site (damage of dam crest and concrete slabs).
- Mass movement into reservoir (impulse wave causing overtopping of dam).
- Water waves in reservoir (damage of riprap at the upstream face of ECRDs, local destabilisation of reservoir slopes).
- Temperature effects (cracking of concrete slabs and corrosion of reinforcement).
2. Man-made hazards:
- Design errors, low quality construction works and lack of or faulty maintenance, etc.
- Terrorism and acts of war.
3. Project-specific hazards:
- Dam geometry and layout of face slab with plinth.
- Ageing of face slab (cracking and corrosion of reinforcement) and waterstops.
- Effect of inelastic static and seismic deformations of the dam body on in-plane stresses in the face slab, taking into account the arrangement and detailing of the joints.
- Temperature effects on face slab especially in drying-wetting zone of the face slab.
- Permeability of rockfill and vulnerability to internal erosion, etc.
- Physical degradation of rockfill, collapse settlement, stiffness of rockfill, etc.
- Modifications in dam body and repair works.
4. Site-specific hazards:
- Topography and geometry of dam body.
- Geology (permeable rock, clay interfaces in foundation, karst, etc.) and local site conditions (soil cover, liquefiable material, etc.).
- Seepage in foundation and arrangement of grout curtain or cut-off wall.
- Effect of changes in the ground water table due to reservoir impounding on slope stability, weakening of rock or causing differential foundation movements, etc.
This list is quite exhaustive. Item (3) is mainly related to CFRDs and the face slab, whereas the other items apply to both CFRDS and ECRDs as well as to other types of embankment dam. However, these hazards – many of which are time-dependent – have different effects on CFRDS and ECRDs. In general, the safety implications of these hazards for ECRDs and other conventional embankment dams are quite well understood, but the long-term behaviour of CFRDS and other new types of dam is less well understood as they may not yet have experienced extreme or unusual events. Therefore, in future some dams may have to be upgraded and the design and safety criteria, etc. must be updated. These periodic safety reviews are standard practice in the dam industry. They also include the time-dependent effects of climate change hazards.
It is not the intention to discuss each of the above hazards and its effect on the safety of the face slab of CFRDs as every dam project is unique since in terms of dam safety the priority order of hazards varies.
The main safety aspects of the concrete face discussed in the subsequent sections are as follows:
- Observed damage in face slabs.
- Different deformational behaviour of rockfill and the reinforced concrete of the face slab.
- Detailing of joints in the face slab.
Different hazards may contribute to these items.
Because earthquakes may produce the largest stresses and deformations in all types of dams, emphasis will be put on this extreme load case in the subsequent sections. The seismic load combination also includes the usual static loads such as dead load, water load and silt load. Therefore, if a dam is structurally safe for the seismic load combination, it will also be safe for the static loads. This also applies to CFRDs. Wieland (2010) and Wieland and Brenner (2007) have discussed different seismic safety aspects for CFRDs. The present paper is basically an extension of these papers as new information has become available and several high CFRDs have been built or are under construction. In China, there are plans to build CFRDs with heights up to 300m, in which seismic performance and safety aspects will play an even greater role in the future. Extreme man-made effects such as terrorism and acts of war are not discussed. Face slabs could be damaged by such actions quite easily.
For the safety assessment of dams, the extreme seismic load combination must be analysed. This means that the recent failures of old water storage dams in the US and tailings dams in Brazil and Australia, which were attributed to static liquefaction, would never have been declared safe if the extreme seismic load combination had been considered in the safety analyses.
Observed damage of face slabs of CFRDs and damage analysis
Damage to face slabs has been observed at different CFRDs, mainly for very high dams. The main reasons for the damage, which also caused an increase in the seepage through the dam body, may be summarized as follows:
- Leakage of joints in the face slab due to inadequate joint detailing and installation of waterstops.
- High in-plane stresses in face slab due to deformations of rockfill causing damage of joints and cracks in face slab, when the biaxial strength of the reinforced concrete slab is exceeded.
- Impact damage of slab elements close to abutments due to rockfall.
- Shrinkage and thermal cracks in concrete slabs resulting in accelerated corrosion of reinforcement in face slab.
Typical damage along vertical and horizontal joints in the face slab of the 156m high Zipingpu CFRD in China was caused by the ground shaking during the magnitude 8 Wenchuan earthquake of May 12, 2008. The concrete spalling along the vertical joint was the result of large normal stresses and the detailing of the joint. It is interesting to note that the joint damage is all along the joint despite the fact that the thickness of the concrete slab increases linearly from the top to the bottom of the dam. This means that the high compressive stresses in the face slab are almost independent of the slab thickness. This is typical for structural elements subjected to imposed deformations like, e.g., restrained temperature strains.
The damage to the horizontal joint with the vertical offset and the overthrust displacement is due to the differential movement of the slab support caused by the deformations in the rockfill, which cannot be prevented by the face slab.
The basic structural function of the face slab is to transfer and distribute the water load over a larger area and to compensate for any variations in the stiffness of the rockfill support. Therefore, for the hydrostatic and hydrodynamic loads the face slab will experience some bending moments due to nonuniform rockfill deformations. These bending moments are rather small and will cause cracks as in reinforced concrete structures. In order to ensure watertightness the crack width should be less than 0.1mm. This is a serviceability limit state problem and not a strength problem.
All the visible shrinkage cracks in the face slab of the 182m high Nam Ngum 2 CFRD in Laos were repaired to minimize any leakage through the face slab and to protect the steel reinforcement from corrosion. Steel reinforcement was provided at the top and bottom of the concrete slab. The development of a shrinkage crack can be avoided by using fibre reinforced concrete for the face slab, which increases the tensile strength of concrete.
Discussion and analysis of damage mechanisms of face slabs
The observed damage to the face slabs above the reservoir level was the result of high in-plane stresses and was not caused by the water load. These stresses are due to the different deformational behaviours of the rockfill and the concrete slab. The modulus of elasticity of rockfill may be of the order of 100 MPa, whereas that of concrete under seismic action may be 30-40 GPa, which is a factor of 300 to 400 times larger than that of rockfill. Therefore, if the rockfill portion of the dam deforms, it is partly restrained by the face slab and thus the shear stresses are mobilized along the rockfill-face slab interface. The shear stresses are limited by the friction between the face slab and the rockfill, which depends on the normal stresses due to the dead load of the face slab and the water load. The friction forces are relatively small for the face slabs above the reservoir level and increase almost linearly with increasing water depth in the submerged part and therefore the in-plane stresses will be much larger in the submerged part of the face slab than in the part above the reservoir level.
The in-plane stresses in the face slab are caused by the deformations of the rockfill support and such stresses are also known as stresses due to imposed deformations, similar to temperature, shrinkage and creep strains. These imposed strains can be released by joints or cracks, Therefore, if an adequate joint spacing and joint width between adjacent concrete slabs is provided, these strains can be reduced significantly. Therefore, if concrete spalling is observed along joints and cracks in face slabs, it can be assumed that the joints are closed and compressive stresses can be transferred from one slab to the other, i.e. the face slab acts almost as a monolithic plate subjected to compressive stresses. In the case of tension, the joints will open.
Usually, the selection of the joint width is based on the assumed static deformations of the body of the rockfill dam, which along the crest provide tension zones near both abutments and compression in the centre of the crest. To minimize in-plane stresses due to such deformations the joint width in the compression zone should be increased. This concept works for static deformations, i.e. dead load and water load, however for seismic action the joints can be closed or open because of the oscillatory nature of the ground motion. Therefore, dynamic compressive stresses will occur even in static tension zones when the joint is closed. These compressive stresses may damage the joints when they reach the values of the compressive strength of concrete, which are of the order of 30 MPa. Cracks may also develop when the biaxial strength of the face slab is exceeded in zones with principal stresses in tension and compression rather than under biaxial compression. Inclined cracks are due to high shear stresses.
If cracks develop or a joint is damaged due to high compressive stresses, then in the case of imposed deformations or seismic action the other parts of the face slab and the other joints will be protected from similar damage. This is also an observation made when tensile cracks are formed in concrete dams due to seismic action.
Therefore, in order to protect the face slab from damage, it is recommended that the width of the vertical joints is determined in such a way that they will not be closed under seismic action. For this purpose, a three-dimensional dynamic analysis of the CFRD is required.
As the slopes of CFRDs are usually much steeper than those of ECRDs, the seismic deformations in the crest region of CFRDs (horizontal displacements) are much larger than in other types of embankment dams of similar height and with much flatter slopes. These deformations may create further damage in this part of CFRDs, although this has not yet been observed; but up to now only few CFRDs were exposed to strong ground shaking.
Consequences of damage to the face slab of CFRDs
The consequences of damage to face slabs, i.e. joint leakage and leakage through cracks in the face slabs, are an increase in seepage through the dam. The amount of leakage depends, on the one hand, on the crack width and the hydrostatic pressure, which is maximum at the base of the dam, and, on the other hand, on the seepage through the joint system.
Leakages as high as several m3/sec were observed in dams with damaged face slabs or defect waterstops. For example, for the 92m high Vajiralongkorn (Khao Laem) CFRD in Thailand the maximum seepage was 2m3/sec and for the 202m high Campos Novos CFRD in Brazil the seepage reached 11m3/sec.
In the case of Zipingpu CFRD, which suffered some face slab damage, the maximum seepage depends on the reservoir level and was about 50 l/s for the maximum reservoir level. At the time of the earthquake the reservoir volume was only about 30% of that at the full supply level. Therefore, before the earthquake the seepage was about 10 l/s, which increased shortly after the earthquake to 19 l/sec. This means that the damage caused in the submerged lower portion of the dam was minor.
In general, leakage through the cracked face slab and joints should not be a dam safety issue, but there are cases where excessive leakage has caused severe safety problems. One of the problems may be the use of low quality erodible rockfill.
Damage to the face slab may be higher near the crest due to increased seismic deformations in this zone. Special measures may be required, but the most effective means is by providing flatter slopes of the CFRD. In highly seismic zones the slopes could be 1:1.5 or even 1: 1.6, which is much flatter than a value of 1:1.3 used in zones of low seismicity. These high crest deformations are also a problem of other types of impervious elements (e.g., asphalt cores or concrete core walls), when slope failures near the crest could occur.
Conclusions
The main conclusions of the discussion of different safety aspects of CFRDs and their comparison with ECRDs are as follows:
- CFRDs are vulnerable to overtopping like all types of embankment dams. There are several reasons for overtopping of dams such as inadequate spillway capacity or the malfunction of spillways and/or low-level outlets.
- The face slab is vulnerable to deformations of the dam body, e.g. due to settlements after construction of the face slab and due to seismic deformations. Such deformations may cause cracking in the face slab or damage to vertical (and horizontal – if any) joints. To minimize deformation of the rockfill, it must have a high modulus of elasticity, i.e. rockfill must be well-compacted and have a proper grading of the particles.
- It must be checked, if face slab leakage may create safety problems to the dam. Fine material could be washed out causing further dam deformations. In order to minimize deformations of the dam body, the rockfill must be well compacted and must have a high modulus of elasticity (or deformation modulus), which can only be achieved by well-graded rockfill, i.e. there must be particle breaks during compaction. Rockfill particles with very high strength like basalt or some other types of rock with uniform particle size will result in rockfill of low modulus of elasticity of, e.g., less than 50 MPa, whereas for high CFRDs values exceeding 100 MPa are needed. The breakage of rockfall pieces could mean that the rockfill will not drain as quickly as assumed in the design and could lead to a small rise in water level within the rockfill depending on the amount of leakage through the face slab.
- The face slab, joint sealing and plinth may be exposed to falling rock near the abutments, and any damage to them may cause local seepage in that part of the dam.
- The face slab (near the crest) could also be damaged by explosives or the impact of boats.
- CFRDs could be damaged by strong earthquake ground shaking as in the case of the Zipingpu CFRD in China, during the 2008 Wenchuan earthquake. The detailing of the vertical joints has a major impact on the damage to face slabs under both static and seismic deformations. As the slopes of CFRDs are much steeper than those of ECRDs, the seismic deformations in the crest region of CFRDs (horizontal displacements) are much larger than in other types of embankment dam of similar height and with much flatter slopes. These deformations may create further damage in this part of CFRDs.
- CFRDs are vulnerable to fault movements in the footprint of the dam and also to non-uniform foundation settlements, especially when the dam is not built on rock.
- CFRDs may have a shorter lifespan than ECRDs, due to the corrosion of the reinforcement in the relatively thin face slabs. Ultimately, the reinforcement will start to corrode despite a concrete cover of the reinforcement of say 7-10 cm. Most vulnerable are cracked slabs and slabs near the crest, which experience several wetting-drying cycles or even frost cycles.
It may be concluded that face slab leakage is the main safety concern and there is a need to evaluate the effect of this leakage on the safety of the dam body, taking into account that the permeability may be substantially less than assumed in the design.
This article first appeared in International Water Power magazine.